New Inroads into Combating Diseases Such as Diabetes and Cancer: Balancing IL-1 Activity with Targeted Therapy.
Interleukin-1b (IL-1b) mediates a broad spectrum of acute and chronic interleukin-auto-inflammatory processes. The cytokine activity is highly regulated. Importantly, chronic IL-1b mediated inflammation is recognized as a major contributor to an expanding list of diseases ranging from depression to heart failure, diabetes, arthritis and cancer. Active interest in targeting IL-1b in cancer comes from the successes in treating multiple myeloma patients who are at risk of advancing to overt disease. Significant reduction in cancer progression is accompanied by treatment with IL-1 blockade which lacks the toxicity of traditional chemotherapy. In a paradigm shift from PPARg targeted therapies, attenuation rather than abolishment of IL-1b activity is a promising strategy to both improve glucose tolerance and stimulate b-cell regeneration in type-II diabetes. We will exploit our ability to convert IL-1b into an antagonist and IL-1ra into an agonist by systematically altering regions with “geometric frustration” to create a series of mutant proteins with a range of inhibitor/signaling activities. Given the risks of contracting life-threatening infections with total IL-1 blockade, attenuation of activity with engineered partial agonists/antagonists is central to current drug discovery efforts (Figure1).
Folding-Function Interplay in IL-b: Specific Mutations Can Impart New Function to the IL-1ra/IL-1β scaffold.
IL-1ß is the only cytokine agonist that is known to have a naturally occurring antagonist (IL-1ra). Both proteins belong to the ß-trefoil family and compete for binding to the extra-cellular IL-1 receptor (IL-1R- Figure 2). Thus, IL-1ß and its natural antagonist, IL-1ra, have coevolved to tightly regulate the activity associated with IL-1 receptor signaling. Motivated by insights gained from our theoretical studies of hybrid IL-1β/IL-1ra structures we expanded our research efforts into the biological investigation of IL-1 mediated receptor signaling. IL-1β/receptor crystallographic data indicated that insertion of the IL-1β functional β-bulge into the tight turn of IL-1ra would introduce agonist activity. Instead, antagonist activity was retained. However, using experimental data from our laboratory, we predicted that mutation of a site in IL-1ra diametrically opposite to the receptor binding site had potential for allosteric regulation of activity. Based on Nf-κB reporter assays, our prediction is borne out (Figure 2). Strikingly, the replacement of C116 in IL-1ra confirms the allosteric potential we predicted for this site and results in agonist activity despite the distant location of C116 relative to receptor interaction sites (blue spheres, Figure 2). In addition, mutation of the structurally homologous residue in IL-1β (diametrically opposite to the receptor interface) results in inhibition of signaling (unpublished results). We are analyzing the theoretical/experimental folding landscapes to determine if perturbation of contacts with residue 116 results in any variation in the folding routes and in relative transition state barriers relative to the parent protein (IL-1ra or IL-1β). We specifically are asking- Has the observed folding route changed? Is an intermediate still populated along the folding route? If so, what is the relative stability of this species? How do structural intermediates (if populated) compare to that observed for the IL-1β and IL-1ra proteins? Are the changes in activity limited to these sites and/or specific mutations? Can we define additional allosteric areas of the functional crosstalk that lead to agonist/antagonist activity in IL-1β and IL-1ra, respectively. If there are differences between experimental and theoretical results, can expanded all-atom models account for the observed differences?
Folding-Function Interplay in IL-b: Route Switching on the Agonist/Antagonist Landscape.
IL-1b populates a well-defined structured intermediate on the folding pathway in which the central strands form early followed by packing of the N- and C-terminal strands to close the b-barrel. We replaced residues 48-57 of IL-1b with the three-residue turn region from the structurally homologous antagonist, IL-1ra and then assessed the effects of this functional mutation on the stability, structural integrity, and folding kinetics of the protein. Equilibrium unfolding/refolding and NMR data indicate that deletion the β-bulge and replacement with a turn has minimal effects on the overall stability or global fold of the native-state. A comparative kinetic folding analysis of the structure-based models for both WT and IL-1bΔB show the b-trefoil barrel (as denoted by the formation of the contacts between the N and C terminal strand contacts and other long-ranged contacts) is formed significantly later in WT-IL-1b as compared to IL-1bΔB (Figure 3A). Figure 3B shows the first 5 seconds of the refolding reaction for WT (black) and IL-1bΔB (cyan). Despite equivalent thermodynamic stabilities, the functional-deletion clearly demonstrates faster kinetics in unfolding (lower right) and the early phase of refolding (Figure 3C, 7D), as compared to the WT. These data suggest that while the overall native state thermodynamic stability is the same, the kinetic landscape has been significantly altered.
In an effort to determine if the functional deletion alters the nature of the intermediate species as predicted from simulation and suggested by the folding kinetics, we pursued a structural characterization approach. Site-specific details of folding can be captured by rapid quench hydrogen-deuterium exchange (HDX) pulse labeling experiments where one can follow the time course of backbone amide proton protection during refolding. Figure 4 depicts the residue-specific detail captured by HDX pulse labeling and NMR analysis on ribbon diagrams of WT and IL-1bΔB proteins under our current conditions. Both proteins have a similar subdomain of early protection, which is correlated to the central trefoil subunit (b-strands 5-7) (Figure 4, blue b-strands) containing the proposed ‘hydrophobic zipper’. Strikingly, there are key differences in the folding routes populated for these two proteins. WT-IL-1b closes the barrel late in folding (Figure 4A, lower right, red b-strands). Conversely, IL-1bΔB closes the barrel via early protection of the terminal-ends, b-strands 1,4, and 12 (Figure 4A & B, upper left, green b-strands). The glaring structural differences in folding routes between proteins are clearly evident when the strands showing early protection are mapped back onto a contact map (Figure 4B). Early protection of the terminal-ends for IL-1bΔB is consistent with the ends-together route, and early collapse of the central region for the WT IL-1b is consistent with the direct-route as predicted earlier. These preliminary data provide the foundation for our studies probing the question whether changes in function (agonist/antagonist) are related to changes in function of the IL-1 family of proteins.

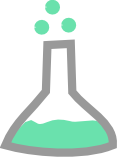